Polycrystalline materials

Polycrystalline materials are common in nature and find applications in many different areas of science and technology. Grain boundary defects (i.e. interfaces between crystal grains) play a key role in determining many materials properties but are in many cases poorly understood. To help address this important problem we are developing first-principles based computational methods to predict the atomic-scale structure and properties of grain boundary defects. Working closely with experimental collaborators (Prof. Ikuhara, Univ. Tokyo) we have helped reveal the structure of grain boundaries in a wide range of materials (including MgO, HfO2, Si and TiO2) with relevance to applications in microelectronics, spintronics, catalysis, surface science and electron transport in solar cell materials.
Metal-oxide electronics

Metal-oxide materials are at the heart of a number of current and emerging device technologies with applications in areas such as logic, data storage, optoelectronics and sensing. Atomic-scale defects in oxide materials are often detrimental to performance but in some cases can be essential for realising functionality. We are developing and applying approaches to predict the properties and dynamics of defects in oxide materials in order to drive improvements in device performance. For example, in recent work we have helped understand the resistive switching effect in HfO2 which can be used for a non-volatile and energy-efficient memory technology. We are also investigating the role of defects in oxides such as Fe3O4 and MgO with relevance to spintronic device applications.
Electron trapping

In many materials atomic-scale defects such as vacancies, interstitials and impurities are able to trap electrons and therefore play a critical role in determining electronic, chemical and optical properties. In materials with large electron-phonon coupling electrons can even self-trap in the perfect lattice forming small polarons. Such effects underpin a range of fundamental and applied issues including superconductivity, magnetism, electron transport in semiconductors and photocatalysis. We are employing a range of self-interaction corrected quantum-mechanical approaches to accurately model electron and hole trapping in nanostructured materials (including surfaces and interfaces).
Oxide nanopowders
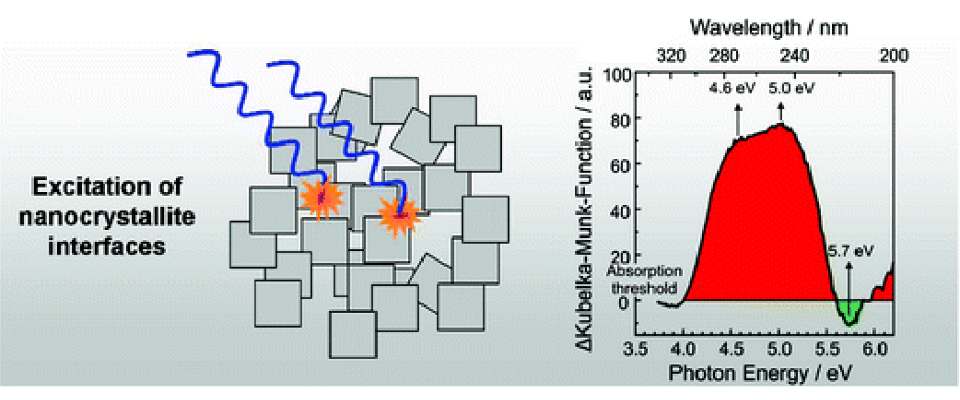
Metal oxide nanopowders comprising a porous network of interconnected nanocrystals represent an interesting class of system with broad applications in chemistry, energy materials, sensing and optical materials. In a long-running collaboration (Prof. Diwald, Univ. Salzburg) we have helped to understand the electronic and optical properties of MgO nanopowders and investigated means to modify them in a controlled way via doping. Our studies have helped elucidate the role of nanocrystal interfaces as charge trapping and luminescence sites and demonstrated the potential of doped MgO nanopowders as luminescent materials for solid state lighting.
Dynamic nanoparticles
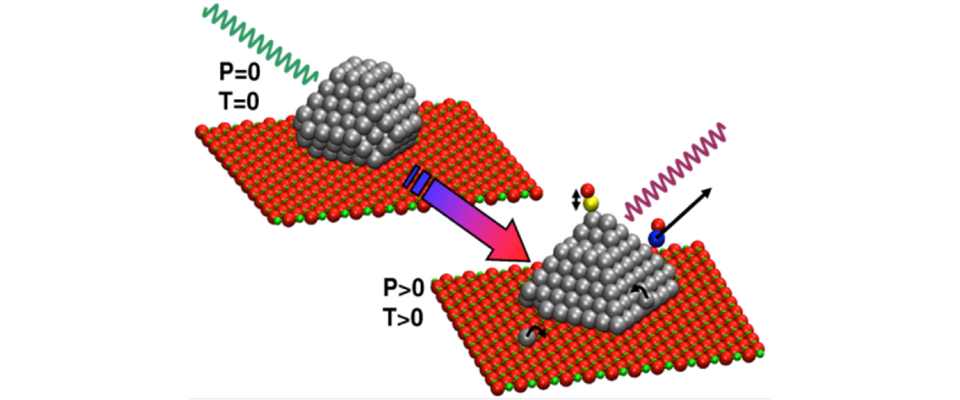
Dynamical interactions between nanoparticles and surrounding molecules can induce both overall morphology transformations and atomic scale fluctuations in their structure. As a consequence properties (optical, electronic and chemical, for example) can be significantly different from those predicted by simple models. This is important for numerous technological applications in areas such as catalysis, gas sensing, and drug delivery, and also for the interpretation of fundamental studies in nanotechnology. We are developing computational approaches to model these effects and recent work has demonstrated how gold naoparticles can be reshaped by adsorption of carbon monoxide molecules.