Heat Assisted Magnetic Recording
Heat-assisted magnetic recording is a potential technology which can enable density increases in next generation magnetic hard disk drives. Current technologies utilise a magnetic storage medium consisting of small magnetic grains, which are bistable and can retain their magnetic state for at least 10 years. The data is written to the storage medium using an electromagnet, which generates a magnetic field as it passes over the spinning medium and changes the magnetic state of the grains.
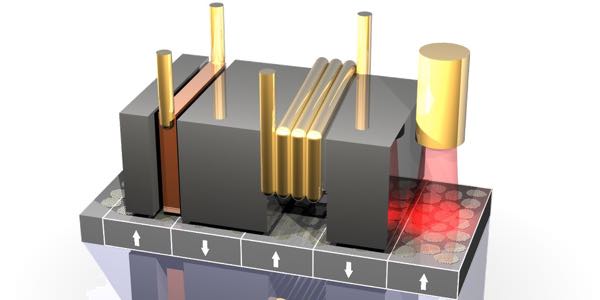
Visualisation of Heat Assisted Magnetic Recording showing granular recording media, write head and laser heat source.
When we try to increase the amount of data in the same physical area a problem arises. To retain sharp bit transitions, where the boundary between the '0' and '1' orientated areas of the media are well defined, the grain size of the medium must be reduced. This then requires an increase in the magnetic anisotropy of the grains, which ensures that the magnetic state is stable for greater than 10 years. However, the magnetic field generated by the electromagnet (or write head) is limited by the material and is theoretically around 2 Tesla. Now, the magnetic field required to reverse the grains depends on the magnetic anisotropy of the material, and so there are conflicting requirements for 'thermal stability' and 'writability'. This problem is known as the magnetic recording trilemma, and this encapsulates the key principles of magnetic media design.
Possible solutions to the trilemma involve changing the properties of the material so that the writability and thermal stability requirements are both satisfied (for example using Exchange-Coupled-Composite Media), changing the material type to a single large grain (Bit Patterned Media), or changing the material properties during the write process. The latter example is where the heating part comes in. Magnetic properties such as anisotropy and magnetisation are temperature dependent, and so by applying heat it is possible to reduce the magnetic anisotropy during the write process, while at the storage temperature the data is stable for > 10 years. This is the principle behind Heat-assisted magnetic recording, or HAMR.
The quadrilemma of magnetic recording
An outstanding question for magnetic disk drives is what is the maximum achievable data density, based on the fundamental physical limitations of the recording medium. As the grain size in magnetic media is reduced, the thermal fluctuations in a magnetic system become much more important in governing how the system behaves. Thermal fluctuations in fine particle magnetic systems are known to cause a loss of magnetic state over time, which is the same phenomenon which gives magnetic recording media its stability for around 10 years. However, for very small systems (and high temperatures) these fluctuations can also cause errors in the writing process, where the applied field is insufficient to guarantee that the individual grains are oriented in the correct direction. This new requirement of 'thermal writability' is then in addition to those of the usual trilemma, requiring that the magnetic moment in the recording media remains high, or for HAMR that the media that the writing temperature is not too high. Hence the trilemma is really a quadrilemma, requiring all four components for a successful magnetic storage system.
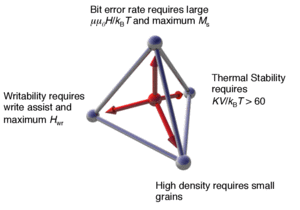
Schematic of the quadrilemma of magnetic recording.
The quadrilemma has implications for the maximum achievable storage density, as now 'thermal writability' becomes the limiting factor in how small the grains can be. Using the ultimate storage medium with a single grain per bit and HAMR to allow writing, the maximum achievable density is around 25TBit/in2, which is considerably lower than one would expect from the trilemma alone.
Related Publications
[1] | Thermally induced error: density limit for magnetic data storage. R. F. L. Evans, R. W. Chantrell, U. Nowak, A. Lyberatos and H. J. Richter Appl. Phys. Lett. 100, 102402 (2012) [PDF] |
[2] | The thermodynamic limits of magnetic recording. H.J. Richter, A. Lyberatos, U. Nowak, R. F. L. Evans and R.W. Chantrell J. Appl. Phys. 111, 033909 (2012) [PDF] |
[3] | Atomistic simulation of sub-nanosecond non-equilibrium field cooling processes for magnetic data storage applications R. F. L. Evans and W. J. Fan Appl. Phys. Lett. 105, 192405 (2014) [PDF] |
[4] | Atomistic modeling of magnetization reversal modes in L10 FePt nanodots with magnetically soft edges Jung-Wei Liao, Unai Atxitia, Richard F. L. Evans, Roy W. Chantrell and Chih-Huang Lai Phys. Rev. B 90, 174415 (2014) [PDF] |
[5] | Atomistic calculation of the thickness and temperature dependence of exchange coupling through a dilute magnetic oxide R. F. L. Evans, Q. Coopman, S. Devos, W. J. Fan, O. Hovorka and R. W. Chantrell J. Phys. D: Appl. Phys. 47, 502001 (2014) [PDF] |